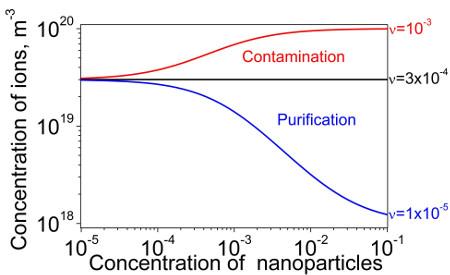
Liquid crystal devices (laptops, cameras, mobile phones, TVs, monitors, etc.) became an important facet of modern society. The future progress in the development of these devices relies on new liquid crystal materials capable to fulfill strict industrial requirements. The combination of liquid crystals and very fine materials called nanoparticles is a “hot” topic in modern liquid crystal science.
Ions which are permanently present in liquid crystals is a serious challenge, the modern display industry is facing nowadays. Even high resistivity liquid crystals characterized by extremely low concentration of mobile ions can get contaminated in uncontrollable way. This uncontrolled contamination can happen at the stage of the device fabrication or while operating the device. Thus, the development of new methods for the permanent purification of liquid crystals from ions is needed.
One promising way to solve it is the use of nanoparticles. It is a well-established fact that nanoparticles in liquid crystals can capture mobile ions and reduce their concentration. A decade long research conducted by independent international teams revealed that the ion trapping scenario is not the only possible outcome of the nanoparticles' dispersion in liquid crystals. While the majority of the published manuscripts report the ion capturing effect of nanoparticles dispersed in liquid crystals (purification regime), there are publications presenting opposite results (contamination regime). (A comprehensive overview of the current state of the art can be found in [1]). A quantitative explanation of these different regimes (purification and contamination) in liquid crystal nanocolloids was lacking.
Recent series of papers [2-6] contributed to our understanding of very complex behavior of nanoparticles in liquid crystals. A common believe is that the ionic purity of nanoparticles is assumed to be 100%. This assumption was called into question in [2]. Instead of being 100% pure, nanoparticles were considered as partially contaminated with ions prior to dispersing them in liquid crystals. The level of this ionic contamination was quantified by means of the dimensionless contamination factor. This assumption combined with classical Langmuir adsorption isotherms resulted in the prediction of three possible regimes in liquid crystals doped with contaminated nanoparticles. These regimes include the purification (decrease in the concentration of ions), the contamination (increase in the concentration of ions), and no change in the concentration of mobile ions. Switching between these regimes is governed by the three dominant factors: ionic purity of liquid crystals, ionic purity of nanoparticles, and the ratio of the adsorption rate of the desorption rate. An example of these three regimes is shown in Figure 1.
Basic idea of paper [2] (this manuscript has been selected as an APL Editor's pick) was developed further in 4 subsequent publications [3-6]. Three papers were published in “Liquid Crystals” [3-5], and one manuscript was published in “Chemical Physics Letters” [6]. These publications demonstrated very good agreement between theoretical predictions and available experimental data. As a result, simple formalism presented in [2-6] can be applied to design very efficient ion capturing nanomaterials for permanent purification of liquid crystals from ions. An important parameter called the ion trapping coefficient is very useful to characterize the ion capturing capability of such nanomaterials. The ion trapping coefficient is positive in the purification regime, and negative in the contamination regime [6]. In the purification regime it equals the fraction of mobile ions trapped by nanoscale materials dispersed in liquid crystals. For instance, if the ion trapping coefficient equals 0.4 then 40% of mobile ions are trapped by nanodopants. Ideal ion trapping nanomaterials are characterized by the ion trapping coefficient = 1. Figure 2 shows an example of ion trapping coefficients of contaminated nanomaterials for different regimes.
Findings [2-6] have very important practical implications for liquid crystal display (LCD) industry. Results presented in [2-6] provide a way to design highly efficient ion trapping nanomaterials for the permanent purification of liquid crystals from ions. The ionic purity of nanoparticles used to purify liquid crystals form ions should be as high as possible. Figures 1 and 2 indicate that even minor fraction of the ionic contamination of nanodopants can reduce their ion trapping capability significantly.
The use of highly purified nanomaterials can lead to the permanent purification of liquid crystals. As a result, ion-related negative side effects such as image sticking, image flickering, and overall slow response of liquid crystals devices can be eliminated. In a long run, totally new driving methods of liquid crystal devices can be developed.
References
[1] Y. Garbovskiy, I. Glushchenko, Nano-Objects and Ions in Liquid Crystals: Ion Trapping Effect and Related Phenomena, Crystals, 5(4), 501-533 (2015).
[2] Y. Garbovskiy, Switching between purification and contamination regimes governed by the ionic purity of nanoparticles dispersed in liquid crystals, Applied Physics Letters, volume 108, issue 12, 121104 (5 pages), 2016.
[3] Y. Garbovskiy (2016): Impact of contaminated nanoparticles on the nonmonotonous change in the concentration of mobile ions in liquid crystals, Liquid Crystals, DOI: 10.1080/02678292.2015.1133850
[4] Y. Garbovskiy (2016): Electrical properties of liquid crystal nanocolloids analysed from perspectives of the ionic purity of nano-dopants, Liquid Crystals, DOI: 10.1080/02678292.2015.1132784.
[5] Y. Garbovskiy (2016): Adsorption / desorption of ions in liquid crystal nano-colloids: the applicability of the Langmuir isotherm, impact of high electric fields, and effects of the nanoparticle’s size, Liquid Crystals, DOI:10.1080/02678292.2016.1145270.
[6] Y. Garbovskiy, Adsorption of ions onto nanosolids dispersed in liquid crystals: towards understanding the ion trapping effect in nanocolloids, Chemical Physics Letters (2016) volume 651 pp. 144-147. DOI: 10.1016/j.cplett.2016.03.050